Understanding Cereal Grains
How the anatomy of cereal grains affects the brewing process—and how to apply this knowledge to gluten-free brewing
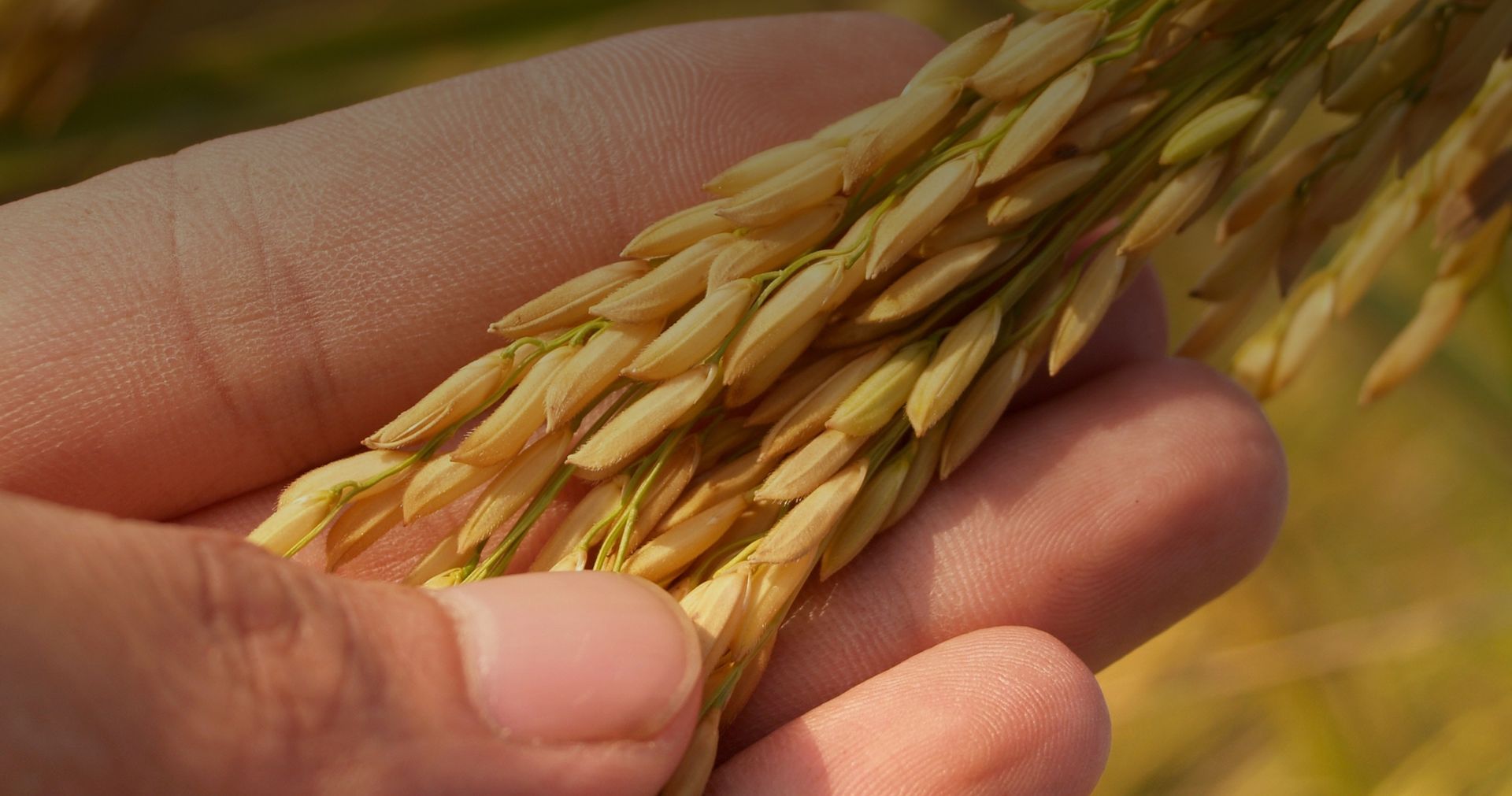
One of the most baffling aspects of gluten-free brewing is that your ingredients don’t always behave consistently between different recipes, or even between the same recipe brewed on different equipment.
I’m only beginning to unravel some of the mechanisms involved, but I have found that having a better understanding of cereal grain anatomy has been helpful in hunting down solutions. There are anatomical features of barley that have gotten baked into traditional mashing advice, but those features don’t always hold true for other grains. By understanding how grains differ from one another, we can come up with better mashing protocols.
Components of a cereal grain
Most cereal grains have four major components: the hull or husk, the bran layer, the endosperm, and the embryo or germ. These layers can be of different sizes and contain wildly different proportions of protein, starch, and fat. For brewing, we tend to favor grains with higher starch and lower oil content, which is why rice and barley are common but sesame and flax aren’t.
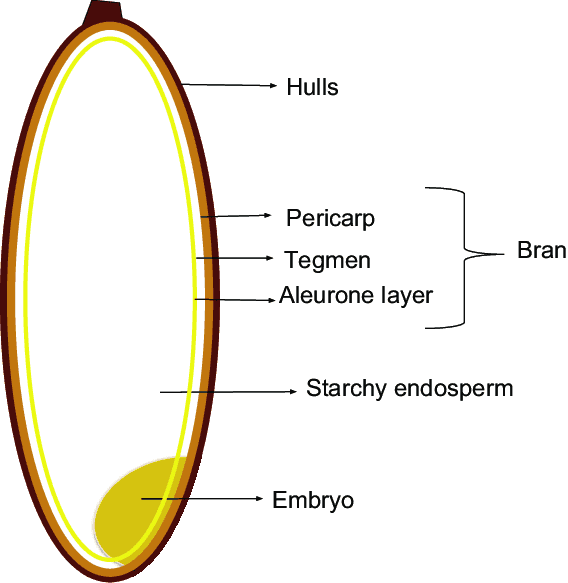
The structure of a cereal grain, as published by Research Gate
Hull: Not all grains have a hull, but when it exists, it is mainly useful to brewers as a filtering aid. Made up largely of indigestible cellulose, hulls are resistant to brewing enzymes, although they do release phenols (including tannins) into the wort, especially if temperatures and pH become elevated.
Bran: The bran layer is a thin coating that holds the lion’s share of the seed’s proteins and fats. The difference between white and brown rice, or whole wheat flour vs. white, is the bran layer. When grains are refined, this layer is rubbed off. From a brewer’s perspective, the bran can be both good and bad, contributing certain essential flavors and wort nutrients but carrying risks if handled improperly.
Endosperm: This is the largest part of the cereal grain and contains most of the starch. It is the portion of the grain that we convert to sugar and then eventually to alcohol.
Embryo: Finally, the embryo or germ contains all of the DNA coding the seed needs to grow into a plant. It is less important to us as brewers, except for the fact that it will be activated by the malting process, causing a range of chemical reactions to take place within the seed.
One side note: you may have heard the term pseudocereal floating around to describe certain grains. This just means the grain comes from a bush rather than a grass. Buckwheat and quinoa are both pseudocereals.
Different types of starch
Starch is, of course, the main cereal component of interest to us as brewers. All starch is made up of chains of glucose, the simplest sugar. Each glucose molecule consists of a ring of six carbon atoms, held together with oxygen and hydrogen molecules, and glucoses can join with each other at specific points in the molecule.
The most common connector is the α-1,4-glycosidic bond, which connects the first carbon of one molecule to the fourth carbon of the next. Long chains of 1,4 bonds create amylose, the simplest form of starch.
However, it’s rare to find natural starches that are entirely amylose. Most of the time, the starch will also contain some α-1,6-glycosidic bonds (carbons 1 and 6), which cause the molecule to branch out in new directions. These branched chains are called amylopectin.
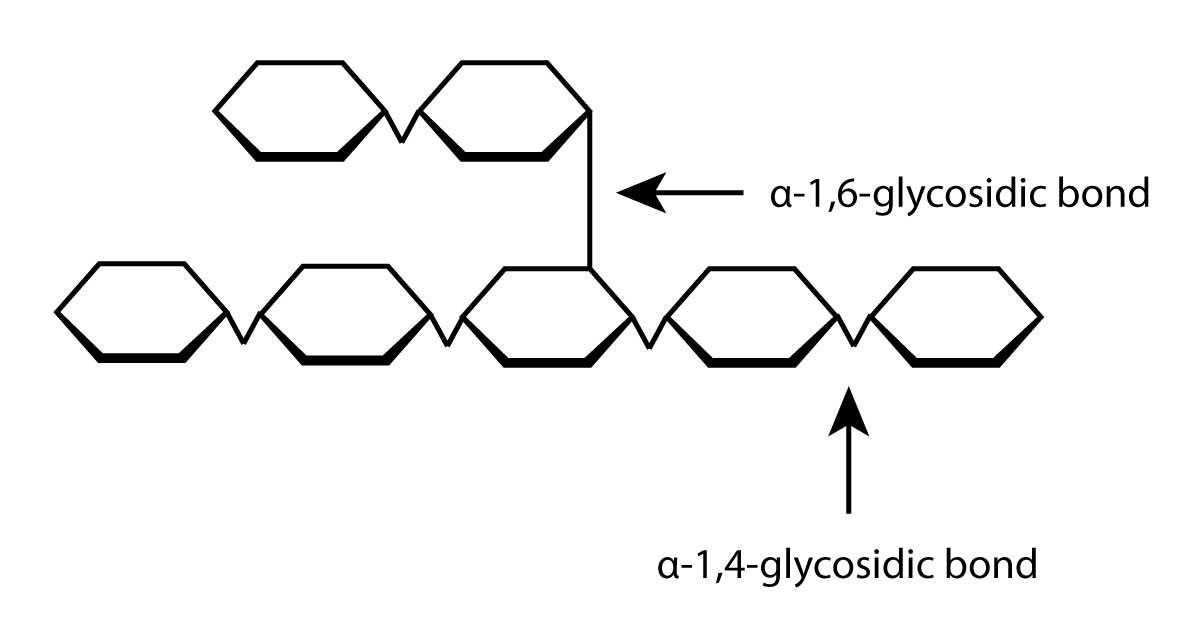
The diagram shows a series of 1,4 bonds and a single 1,6 bond, creating amylopectin.
In most grains, the ratio of amylopectin to amylose ranges from 70% amylopectin on the low side to virtually 100% on the high end. This matters to brewing for several reasons.
First of all, most of the enzymes we use can only attack 1,4 bonds, leaving the 1,6 bonds intact. As a result, molecules consisting of 1,6 bonds are left in the wort as unfermentable dextrins. Of course, most worts are not 70% dextrin, so amylose branches within a larger amylopectin molecule can be broken off and digested by enzymes. However, once the enzymes run into a 1,6 bond, they will stop, leaving behind a limit dextrin (unless a pulllanase or glucoamylase is present).
Secondly, tightly knit amylopectin structures seem to resist mashing altogether, requiring higher temperatures and longer holding times to dissolve into solution. I’ve done a series of mash tests on different varieties of unmilled, whole-kernel rice, and the more amylopectin, the longer it takes to achieve full yield: a long-grain fluffy white rice like basmati gives up nearly all of its starches in under 45 minutes, whereas a short-grain sticky white rice only achieves around 30% in the same time.
The practical impact of this information is that if you use a high amylopectin source, you will need to mill your grain somewhat finer and mash somewhat hotter in order to achieve good yields. Since we as GF brewers are working with a range of cereals that were not bred specifically for brewing, it’s worth it to look into the specific type of cultivar you’re using.
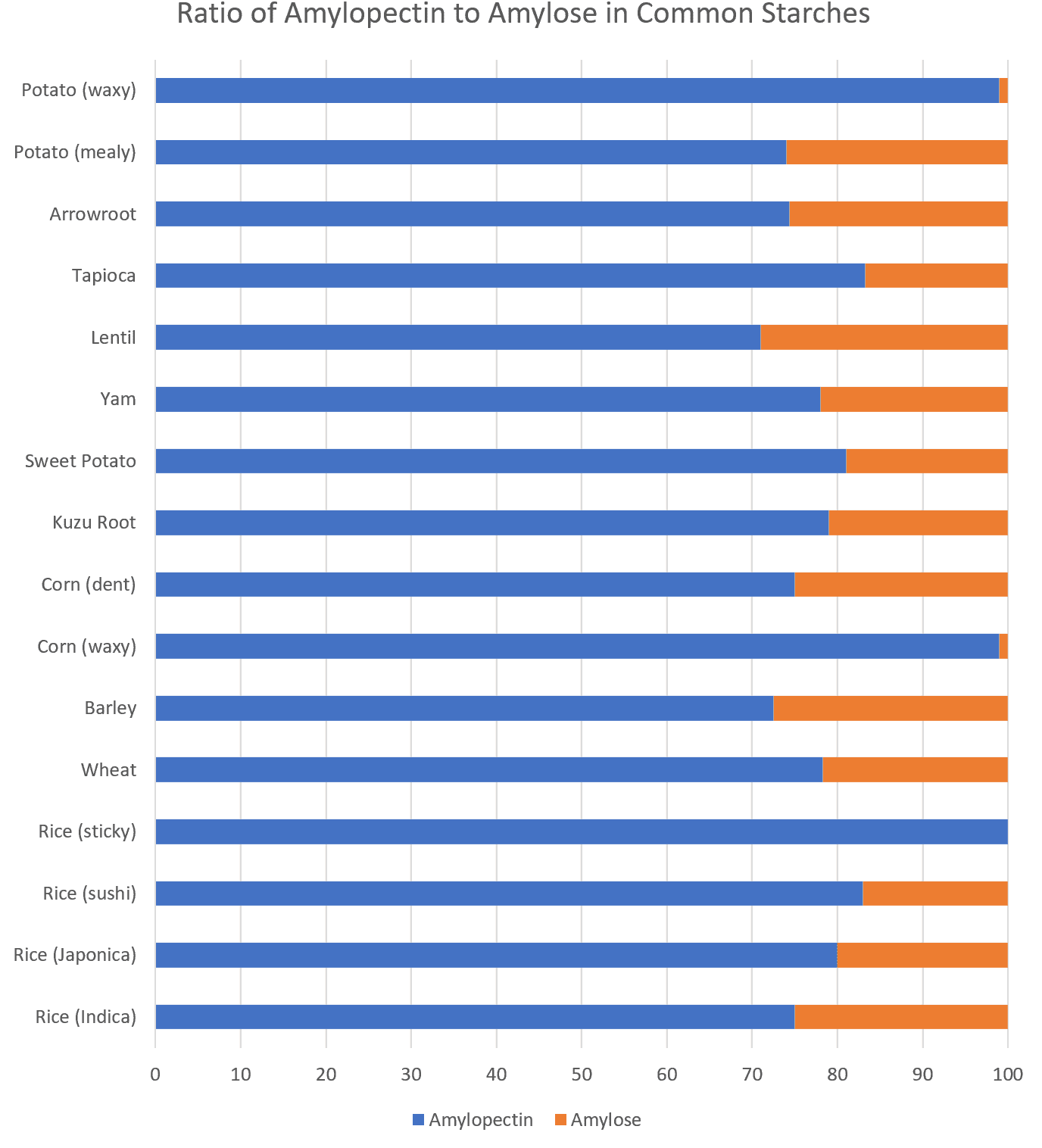
The ratio of amylopectin to amylose in common starches. The blue bar is amylopectin.
Hulls and phenols
The flip side of the amylopectin equation is grain phenols, because the same variables that facilitate starch conversion also facilitate undesirable phenol extraction.
There are thousands of types of phenols, which are essentially aromatic plant compounds, and they can enter your beer at several stages. Brewers tend to think of phenols more in the context of polyphenol hop haze, but in any given beer, gluten-free or not, a majority of the phenolic content comes from the grain hull. Some phenol extraction is desirable to both flavor and appearance, but too much can lead to haze problems, a puckering tannic mouthfeel, and off flavors.
The finer you mill your grain, the more small hull fragments or “flour” you’ll create, exponentially increasing the surface area. This leads to more phenols being extracted into the mash. In addition, the higher the mash temperature and/or pH, the more phenols get extracted. This is the same principle behind tea brewing guidelines: connoisseurs will steep their brews at temperatures below boiling, usually between 170-200°F, to avoid extracting harsh flavors from the leaves.
It’s also important to note that certain proteins will bond with phenols, both in the mash and in the boil, precipitating them out. This is part of the function of the hot break, but these reactions happen in the mash as well. The high protein content of barley seems to make things easier for traditional brewers, since more phenol gets complexed out at both stages. For us, it’s helpful to try to get the clearest wort possible going into the boil kettle to avoid haze problems downstream.
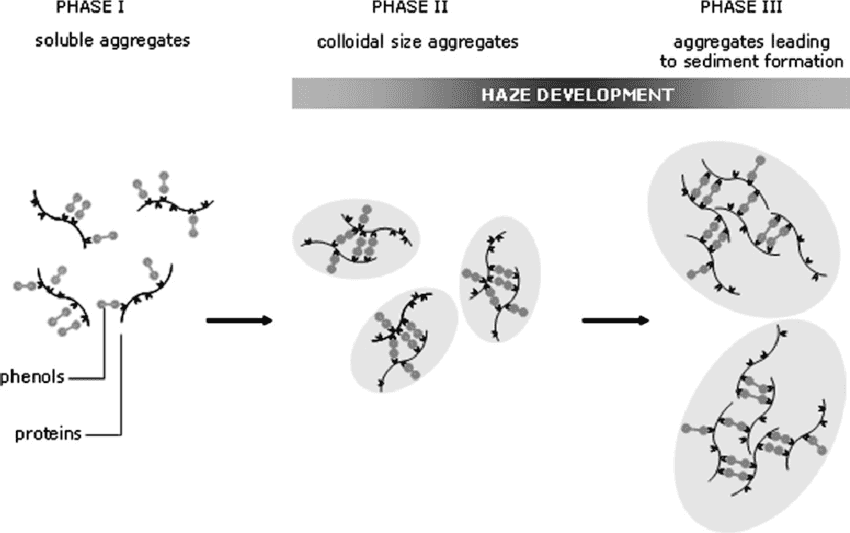
Scheme of polyphenol-protein interaction, as published by Research Gate
It seems that millet-dominant mashes clear out more easily than rice-dominant ones. It’s possible the reason could be that millet has more protein than rice, in which case adding protein via quinoa or some other source might help. It’s also possible millet just releases less phenolic content. I don’t have any definitive data on this, but experimentation along these lines is on my to-do list.
Further complicating the situation, I’ve seen rice-based grain bills with large proportions of biscuit malt clear out better than those without it. I’m not sure what’s going on here either. Maybe the malt roasting process creates conditions that lead to better phenol precipitation, but that’s just a guess.
Regardless, your best bet is to focus on getting good filtration in your mash, avoiding hull “flour,” doing a decently long vorlauf, and transferring your wort to the kettle as slowly as possible.
Proteins and nitrogen
The proteins in cereals play several important roles. They help to complex out phenols, as described above. They also provide nutrients for the yeast, in the form of assimilable nitrogen, and they affect both head retention and mouthfeel.
Most gluten-free grains are lower in total protein than barley or wheat, with the exception of quinoa, and this is the primary reason that head retention can be a problem for us. You can get around this by adding protein, though not all protein is created equal. I’ve tried using chickpea flour, since chickpea-derived aquafaba is used to make the foam in vegan pisco sours, but it didn’t have any noticeable benefit to head retention. On the other hand, a small dose of quinoa or buckwheat seems to go a long way toward adding foam to your beer.
The other side of the protein equation is mouthfeel. My general rule of thumb is that gluten-free beers “feel” about 2°P lower on the palate than their barley equivalents, and the main culprit is here is most likely the lack of protein. To counteract this effect, I usually aim for a slightly higher terminal gravity than I would if it were a barley-based beer. Eventually, I hope to have time to explore other sources of protein and how they might affect mouthfeel and head retention. When that time comes, I’ll post the results here as well.
Role of protein at various stages of the brewing process
Stage | Roles |
---|---|
Malting (or Ondea Pro) | Modify starch content Release yeast assimilable nitrogen and other nutrients Improve lauterability |
Mashing | Precipitate out phenols Release yeast assimilable nitrogen and other nutrients |
Boil | Remove phenols and other undesirable compounds via hot break and cold break |
Fermentation | Nutrition for yeast metabolism Create chill haze by bonding to phenols, then slowly sinking until the beer clears |
Oils and fats
Generally speaking, grain oils are not desirable in brewing and can lead to stale, cardboardy flavors in the finished beer (hop oils are a different story, and it’s a mixed bag when it comes to the oils in various spices and flavorings). The main issue stems from a naturally occurring malt enzyme called lipoxygenase or LOX. LOX is responsible for breaking down fats into their foul-tasting, rancid forms in the presence of oxygen, and a lot of the warnings about hot-side aeration stem from the negative effects of LOX.
The good news for us as GF brewers is that LOX is denatured at about 160°F. So if you start with a high-temperature rest in your mashing process, you theoretically shouldn’t need to worry about hot-side aeration. There may be some LODO brewers out there who will disagree with me here, but whether you splash your wort around or not, it seems like a LOX-denaturing rest is a good piece of insurance.
Outside of the LOX issue, grain fats don’t seem to play a major role in the brewing process, and many of them will eventually settle out in the trub. If you’ve ever brewed a beer with a large proportion of oats, which have about 3x more fat than barley or rice, you will have noticed a larger than usual amount of highly viscous trub in the fermentor. That extra trub volume is due to the fat combining with other wort components and sinking to the bottom.
Cell wall components
Finally, we need a quick word on the non-fermentable cell wall components that break down in both malting and mashing. The most important to brewing are arabinoxylan and ß-glucan, and they are broken down respectively by xylanase and ß-glucanase. Much of the work of these enzymes takes place in the malting process, but they can also play a role in mashing, particularly if you add them in using an enzyme cocktail like Ondea Pro.
In order for your enzymes to reach the starch in each grain, the grains’ cell walls need to be ruptured in some way. Milling your grain is obviously part of this process, but xylanase and ß-glucanase significantly increase access to starches that would otherwise be locked away. Barley brewers have noticed that yield and conversion speed both increase when incorporating a ß-glucan rest into the mash, and there’s no reason to believe this would be any different for GF grains.
In high concentrations, these dissolved cell wall components can also make your wort thicker, which can lead to lauterability problems and haze in the finished beer. They can also impact mouthfeel, which can be a plus or a minus, depending on your desired outcome. For the most part, however, beer characteristics improve as arabinoxylan and ß-glucan are further broken down.
Not all grains release the same amount of cell wall components into the mash. Buckwheat is notoriously high in ß-glucan, which is why buckwheat worts are so viscous and create beers with a slick mouthfeel. In addition, highly modified malts will have had more of their arabinoxylan and ß-glucan broken down during malting than undermodified malts. Raw grains, naturally, will have the most issues with these cell wall components unless extra mashing enzymes are added to break them down.